Outsmarting Heisenberg
Physicists from the Max Planck Society and Leibniz Universität Hannover develop a new concept to improve the sensitivity of gravitational-wave detectors
Laser interferometers detect tiny distance changes with high precision. Stray light reduces and limits the measurement accuracy of these instruments. Researchers at the Albert Einstein Institute in Hannover have now shown for the first time how to discriminate between measurement signal and stray light using lasers with tailored quantum properties. The novel measurement concept circumvents the Heisenberg uncertainty principle and could enhance the precision of gravitational-wave detectors like GEO600 or the closely cooperating Advanced LIGO (aLIGO) detectors in the USA.
Keen quantum eyes for gravitational waves
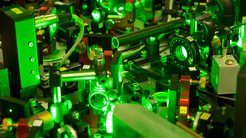
The scientists at the Albert Einstein Institute (AEI; Institute for Gravitational Physics at Leibniz Universität Hannover and Max Planck Institute for Gravitational Physics) in Hannover are hunting for elusive gravitational waves – a prediction from Einstein's general theory of relativity. Their first direction detection will open a new window to our Universe and usher in the era of gravitational-wave astronomy. The AEI researchers aim to detect these tiny space-time ripples using detectors like GEO600 near Hannover, Germany, and aLIGO in the USA. These detectors use lasers to measure the tiny distance changes caused by passing gravitational waves. Thus, the continuous improvement of those lasers and the minimization of disturbances like stray laser light is of great importance.
Now, the AEI physicists for the first time created laser light with tailor-made quantum properties. It even enables them to circumvent Heisenberg's uncertainty principle, which usually limits the accuracy of measurements. “By using our new method we can significantly reduce the disturbances caused by stray light in gravitational-wave detectors. GEO600 would listen for gravitational waves even more sensitively. After a successful integration into GEO600 we can make the technology available to the worldwide network of gravitational-wave observatories,” says Roman Schnabel, leader of the working group for quantum interferometry and squeezed light at the AEI and participating scientist in the research area “Quantum Sensors” in the QUEST cluster of excellence.
Entangled light
plays the main role in the new measurement method developed in Hannover. According to Heisenberg's uncertainty principle, some pairs of quantum mechanical properties cannot be measured simultaneously to arbitrary precision. For particles, this applies to position and momentum, while for light waves, this applies to amplitude and phase.
Until now, the AEI scientists used so-called squeezed laser light in GEO600. They decrease (squeeze) the uncertainty in either phase or amplitude of the light. This comes at the cost of increased uncertainty in the other property. Thus, either the phase or the amplitude can be measured more precisely. “Squeezed light is our instrument of choice for laser-based precision measurements using just one quantum property of light. But we were wondering if there was useful information hidden in the other variable as well,” says Schnabel.
Therefore, the researchers applied another trick. By superimposing two squeezed laser beams, they created two new laser beams which are quantum mechanically entangled. One of the beams is used to perform the precision measurement, the other one is used as a reference beam. By comparing measurement and reference beam, the scientists can now determine both phase and amplitude with decreased uncertainty. This allows them to detect tiny changes in both variables.
“We can now for the first time outsmart Heisenberg's uncertainty principle because we're measuring all variables relative to an entangled reference system,” explains Sebastian Steinlechner, lead author of the study published in Nature Photonics. He is a PhD student in Schnabel's group within the Collaborative Research Centre/Transregio 7.
Showing stray light a red card
In this way, the physicists suppress the disturbance from laser stray light in the detector. Even single laser photons gone astray can combine inseparably with the measurement signal and distort the results. The new method now enables a more precise and independent measurement of both phase and amplitude changes in the laser light. Using this split into two independent components, stray light can be identified immediately during the measurement. Affected data are eliminated from further analysis, thereby increasing the precision of the final results.
The gravitational-wave detector GEO600
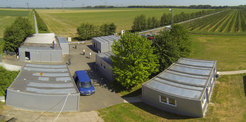
could be the first application for the novel concept. The required technologies have been in use at the detector site for two years and have proven themselves. Since 2011, the measurement precision of the interferometric detector GEO600 has been increased by 50 percent through the use of squeezed laser light.
The sensitivity of the detector, however, can only be increased so far using squeezed light. Ultimately, it can only be further increased by tracking down the stray light. The physicists are confident that they will reduce this disturbance with their new method and thereby increase the probability of the first direct detection of gravitational waves.